Although warehouse fires are hardly being recognised as “hazardous accidents”, they are, in fact, one of the most recurring toxic exposure events. Due to the formation of highly toxic combustion products, these situations often lead to large scale alarming and evacuation actions towards potentially exposed populations.
Warehouses may contain large quantities of liquid or solid combustible products, varying from fertilizers and pesticides to cleaning agents, potentially producing many different toxic combustion products. While we just see a “smoke” plume, it often contains high concentrations of hazardous substances. This smoke plume will initially rise into the atmosphere and carry along wind a series of toxic combustion products that can be harmful to both humans and the environment.
In the figure below, a black smoke plume rising over Haifa’s port is shown, due to a warehouse fire that occurred in 2019.
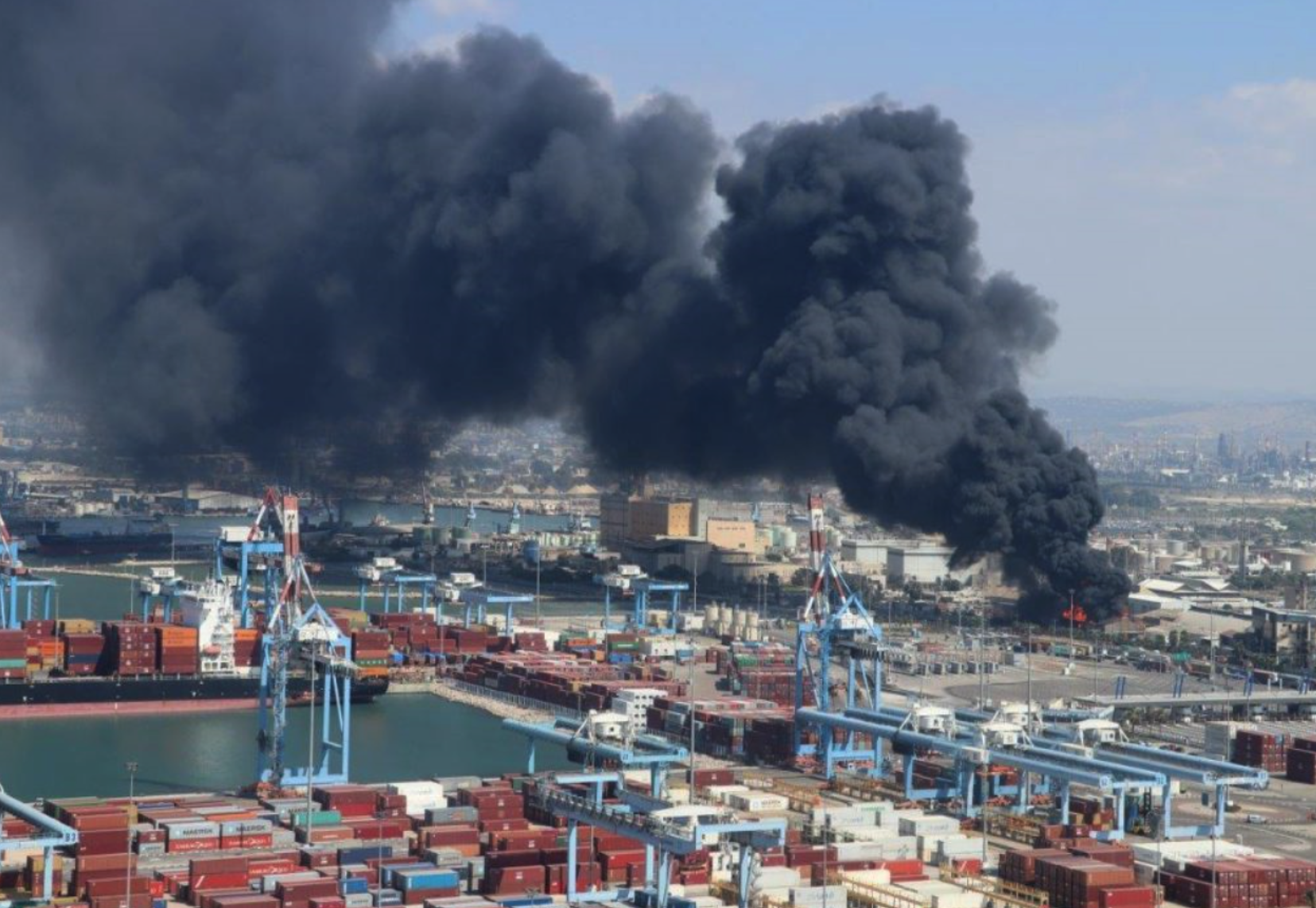
Why modelling the plume rise phenomenon?
Both pool and warehouse fires merely consist of a combustion reaction where the fuel burns with oxygen. Many of the fuels used in the chemical industry are organic hydrocarbons that frequently contain contaminants such as nitrogen, halogens, and sulphur compounds that can result in highly toxic fumes. The resulting toxic combustion products (such as NOx, HCl, HF, SO2, etc.) can reach dangerous concentrations up to large distances, requiring alarming or even evacuation actions by emergency response teams.
That is why there is a growing interest in simulating the effects of rising smoke plumes. The assessment of the toxic concentration levels of these combustion products transported along the plume trajectory is of utmost importance. Safety professionals can use this information for hazard identification, safety analysis and emergency planning.
This is why Gexcon has developed a “plume rise model”, which is implemented in Gexcon’s consequence modelling software EFFECTS. This model calculates not only the trajectory of the plume and the hazard distances to specific concentration threshold levels but also the potential penetration or reflection of the mixing layer height.
Let me explain in detail what the plume rise phenomenon consists of and the penetration and reflection phenomena are.
The plume rise phenomenon explained
Plume rise
Smoke plumes containing toxic combustion products will initially rise due to the density difference between the hot combustion products and the ambient air. This density difference is caused by the fact that the plume temperature is significantly higher than the temperature of ambient air.
The theory behind this plume rise phenomenon foresees that there will be a height at which the finally cooled-down plume will be in equilibrium with the density of the air at that height, leading to a maximum plume height. The trajectory of the plume and the hazard distances to specific concentration threshold levels will be mainly influenced by the wind speed, atmospheric stability class and the fire’s convective heat production. The combination of these parameters leads to potential penetration of or even reflection against the mixing layer.
Influence of mixing layer height
The height of the base of a so-called “temperature inversion layer” from the ground is the mixing layer height. A temperature inversion layer is a layer in the atmosphere that occurs when the air temperature increases with height. In the figure below, you can see the mechanism behind the formation of a temperature inversion layer.
During daytime (see the left picture in Figure 2), the earth absorbs the solar heat radiation and radiates it back to the air near the ground. At this moment of the day, the temperature is highest near the ground. At night time, the air temperature decreases. Because the warm air near the ground now has a lighter density than the density of the surrounding air, this warm air rises, creating a warm inversion layer (see the right picture in Figure 2).
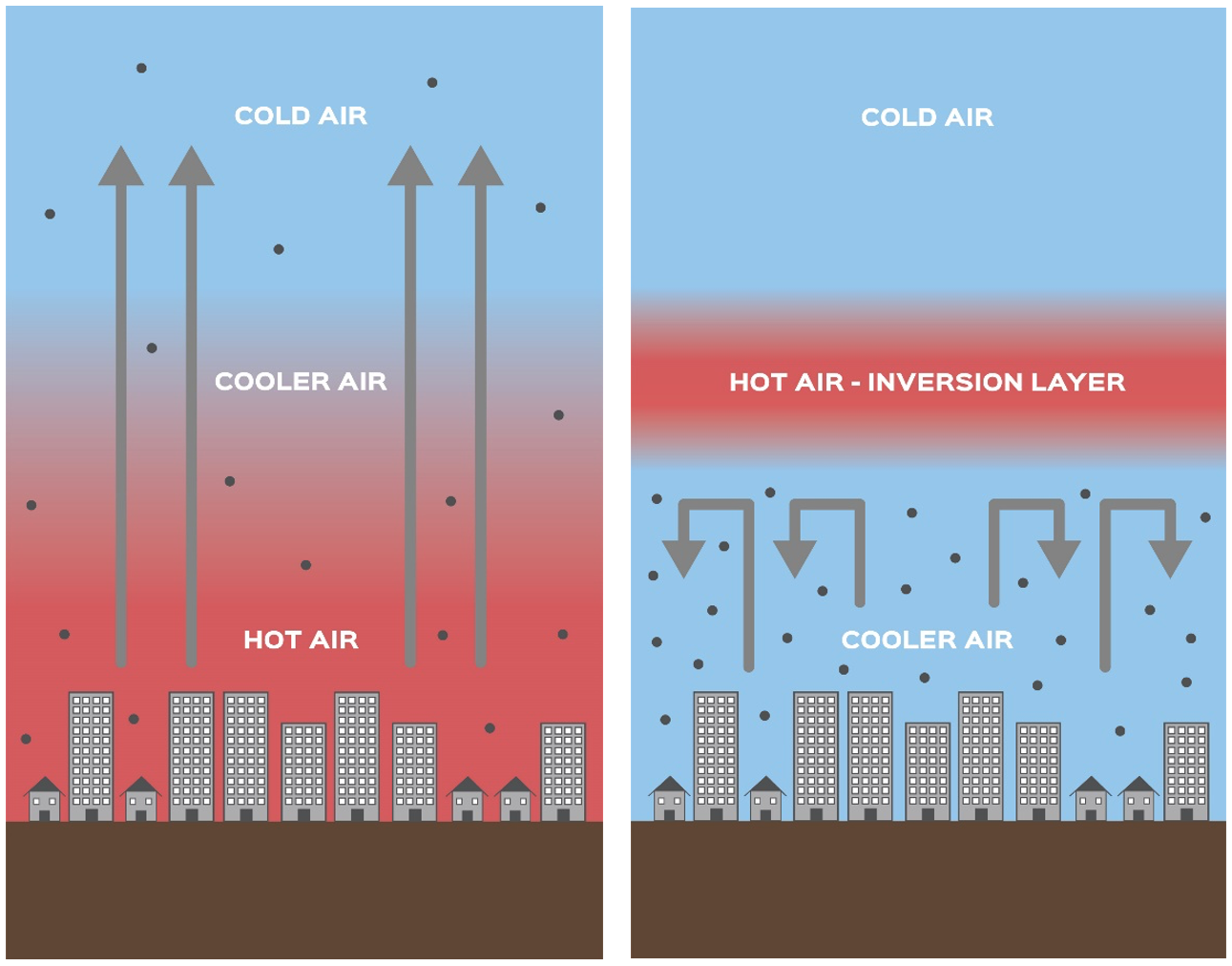
This mixing layer height will act as a ceiling on upward air movement. This is the reason why upon the presence of a temperature inversion layer, pollutants will be trapped under it, as the density difference between the warm inversion layer and the surrounding air retard vertical mixing. Unfortunately, we all know this mechanism too well, as we have air quality alarms in cities because pollutants get trapped in a thin layer above the city (as seen in Figure 3).
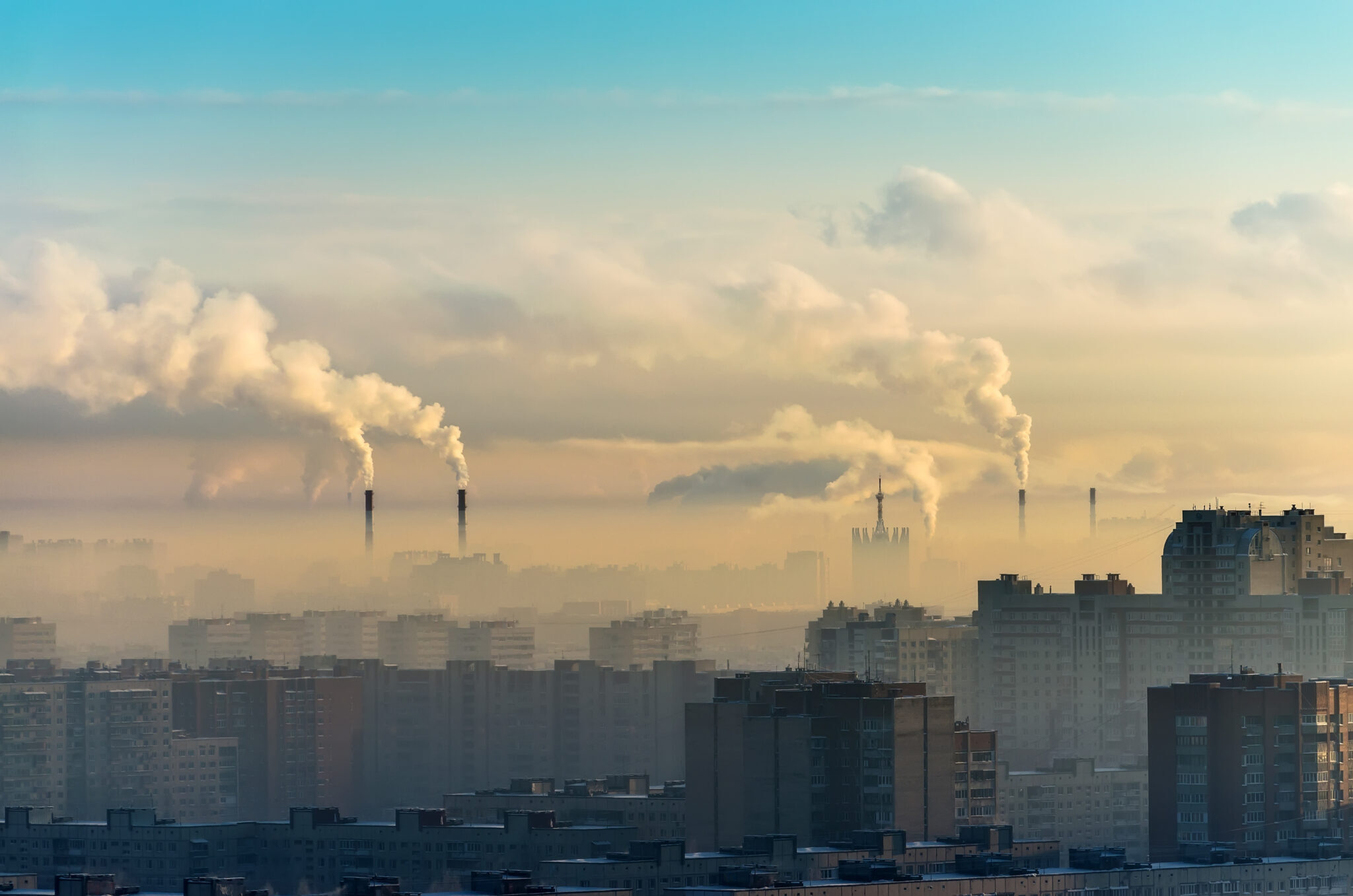
Plume penetration and reflection
Typical models describing the mathematics behind rising hot plumes include the effects of atmospheric turbulence, as described by the Pasquill stability class. However, the plume’s potential penetration of the mixing layer height is often dismissed, which is of great importance to assess toxic exposure of individuals at ground level.
The importance of the plume penetration is that all mass that has risen above the mixing layer will never disperse back into the mixing layer. Therefore, toxic combustion products will be trapped above the mixing layer height and never create toxic exposure at ground level. This is because there is no vertical turbulence at the boundary of the mixing layer (at the temperature inversion height). Only the stronger chimney (hot) emissions are likely to penetrate upwards due to their greater buoyancy forces. Apart from penetration of the mixing layer height, the potential reflection of the plume should also be considered, which especially plays a role for plumes that remain below the mixing layer height. The figure below shows a graphical representation of the penetration and reflection phenomena in smoke plumes.
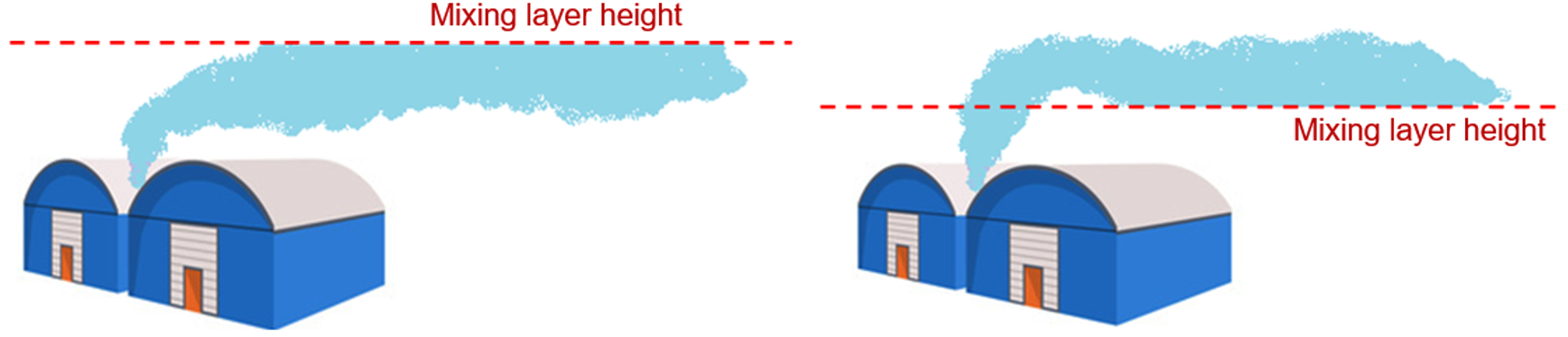
The consideration of the penetration and reflection phenomena is highly relevant when assessing the consequences of a potentially toxic smoke plume. For instance, if the fire brigade is trying to extinguish the fire, that would significantly decrease the heat produced by the fire, leading to a less buoyant smoke plume which might not have enough driving force to penetrate the mixing layer height. Hence, the plume will be trapped below the mixing layer height. That means that trying to extinguish the fire increases the danger of toxic exposure at ground level. Of course, other factors should also be considered such as potential escalation to a bigger and prolonged fire.
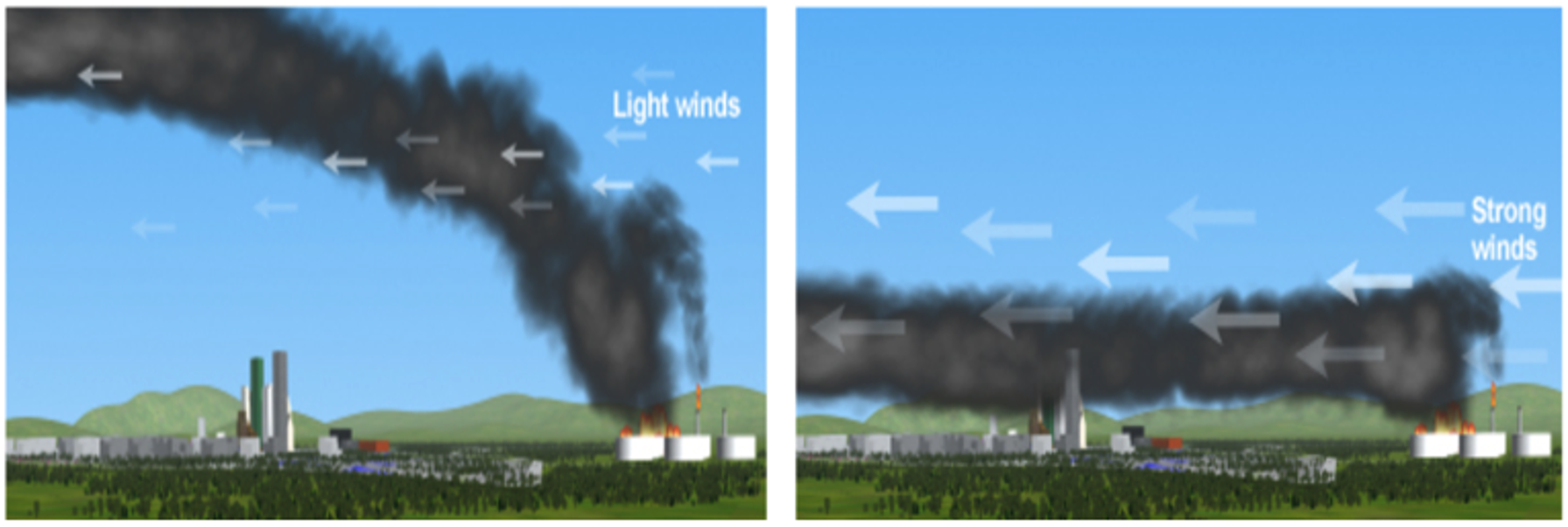
The plume rise model in EFFECTS
The “plume rise model” as implemented in Gexcon’s consequence modelling software EFFECTS calculates the maximum height of the plume, the plume path, and also presents concentration threshold contours of toxic combustion products at any height level.
Unlike any other commercial tool, the “plume rise model” implemented in EFFECTS takes into account the penetration of the mixing layer height (which typically occurs for plumes with a strong convective heat) as well as the reflection phenomenon against the ground and against the mixing layer height (which can occur when the plume is both above and above the mixing layer height).
In the figure below, several EFFECTS results are shown as an example for different smoke plumes.
- The top left image shows a smoke plume fully penetrating the mixing layer height. In this case, there will be no toxic exposure at ground level.
- The top right image shows a smoke plume partly penetrating the mixing layer height. The fraction of the plume that penetrates the mixing layer is trapped above it due to the reflection phenomenon.
- The bottom left image shows a smoke plume that develops very close to ground level and experiences reflection against the ground.
- The bottom right image shows a smoke plume where only a very small fraction penetrates the mixing layer height. The remaining plume develops below the mixing layer height and experiences reflection against it. This forces most toxic combustion products to stay below the mixing layer height, which can potentially lead to toxic exposure at ground level.
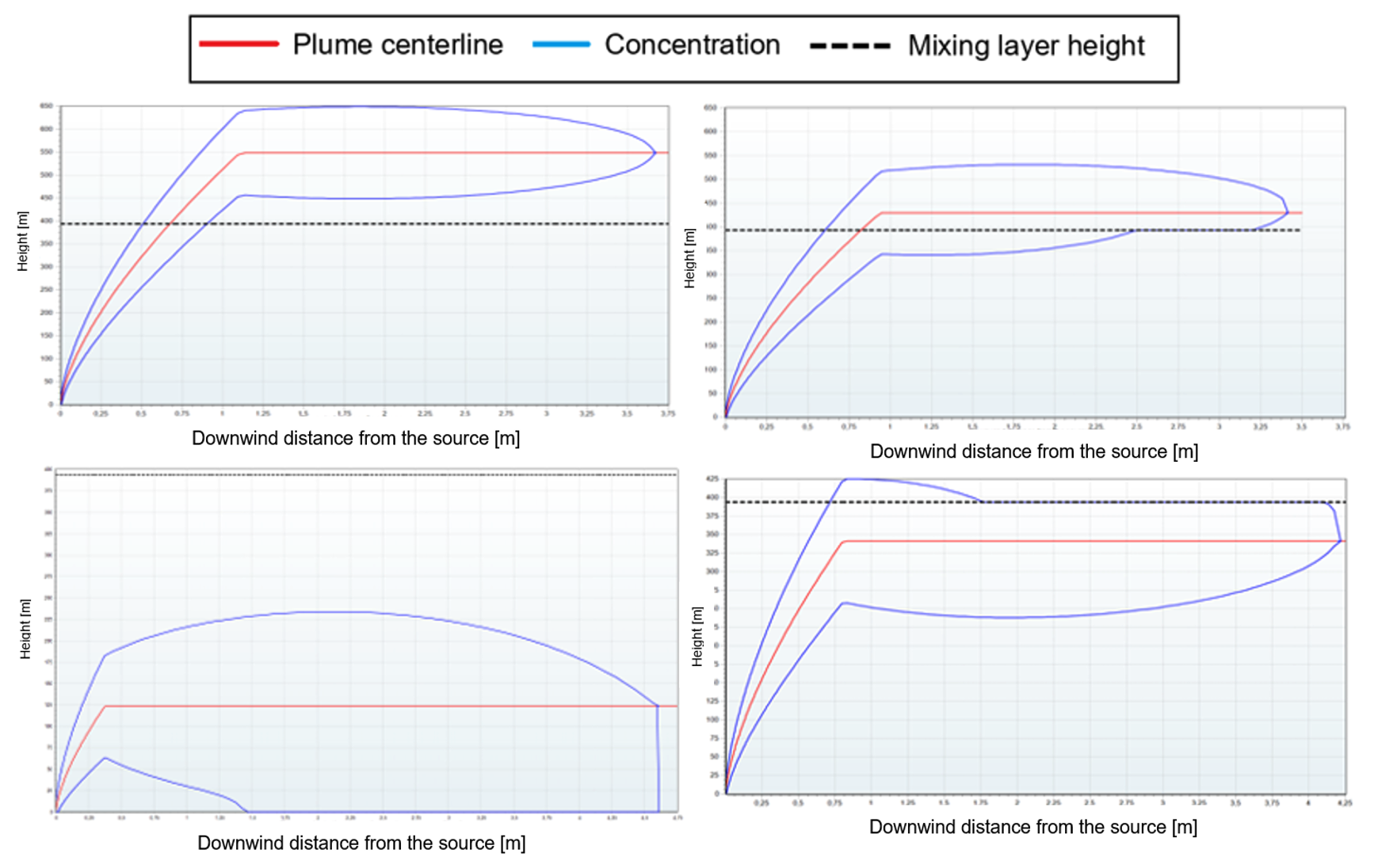
As you can see from the video, simulating smoke plumes due to pool fires and warehouse fires in EFFECTS is very straightforward and user-friendly.
How to use the plume rise model in EFFECTS to calculate smoke plumes
If you want to know how to simulate the plume rise phenomenon in EFFECTS, you can refer to the video below.
Different typical scenarios can lead to the generation of a smoke plume, which can be modelled with the “plume rise model”. The first two cases below are explained in the video. The third example is not explained in the video, but you can find its modelling in the project file included below.
First example
The first example shows how to simulate the full-bore rupture of a loading hose at an airport’s kerosene refuelling station. This catastrophic rupture leads to the generation of a liquid spill, which ignites, forming a pool fire. The pool fire then starts generating a smoke plume due to the incomplete combustion of kerosene.
In this example, the convective heat produced is so high that the plume has enough driving force to penetrate the mixing layer height. As a consequence, individuals at ground level will not suffer toxic exposure from the smoke plume.
Second example
The second example shows a warehouse fire containing several solid combustion materials, which are expressed with an average molecular formula of the contents. The example shows how to simulate the potential effects of a resulting toxic plume from a warehouse fire.
This example shows how different the plume trajectory can be when the heat production is significantly reduced, leading the plume to be trapped below the mixing layer height. This causes the individuals at ground level to be exposed to toxic concentrations of SO2.
Third example
The third example shows how to simulate a smoke plume generated from the direct ignition of a liquid spill of bunker fuel oil containing H2S. During the combustion process, SO2 is generated (among other toxic combustion products), which trajectory can be monitored with the “plume rise model”. In this case, the heat produced by the fire does not give the plume enough driving force to penetrate the mixing layer height. Instead, the plume suffers from reflection and remains trapped below the mixing layer height, potentially leading to toxic exposure at ground level.
In this case, the pool fire phenomenon is calculated to show how the pool’s surface area can be used in the calculation of the generated toxic combustion products. It is assumed that the plume will start at the top height of the pool fire. This is especially relevant for high fires, as the flame height will be the height at which the fumes are actually emitted and have their initial convective heat load.